Design Guidelines: Sheet Metal Fabrication
Sheet metal fabrication is a versatile manufacturing process used to create parts by cutting, bending, and assembling thin metal sheets, suitable for a wide range of industries such as automotive, aerospace, and electronics.
To ensure optimal results, it’s important to consider factors like material selection, thickness, and design for manufacturability (DFM) when designing for sheet metal fabrication.
Size
Maximum Dimensions
Metric | US | |
---|---|---|
Size | 990.6mm x 1,193.8mm | 39 in. x 47 in. |
Bend Length | 1,193.8mm | 47 in. |
Minimum Dimensions
Metric | US | |
---|---|---|
Formed Part | 12.7mm x 12.7mm | 0.500 in. x 0.500 in. |
Flat Part | 6.35mm x 6.35mm | 0.250 in. x 0.250 in. |
Materials
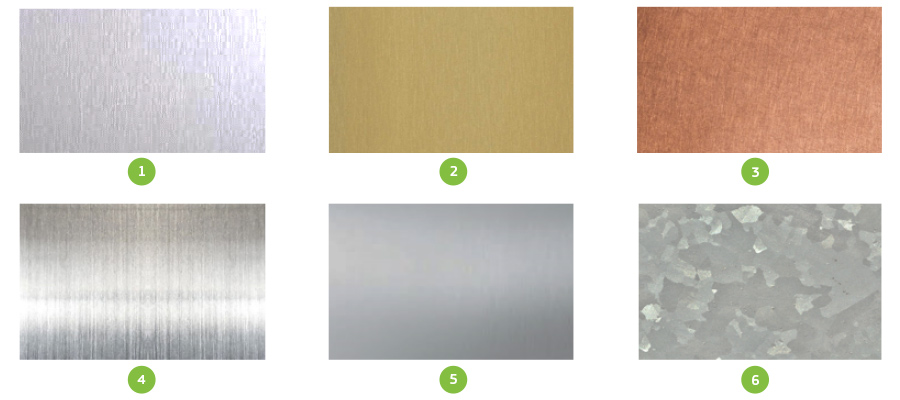
Z Frame
Tolerances
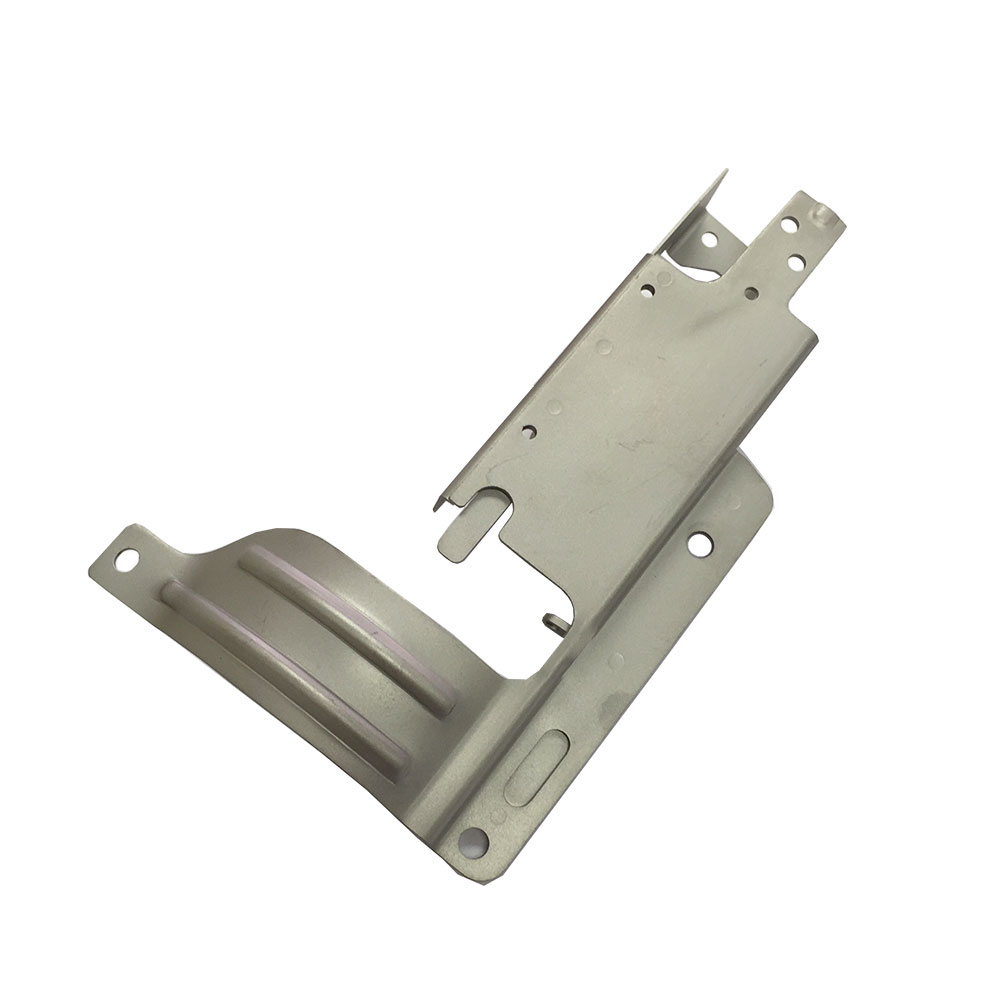
Single Surface
Metric | US | |
---|---|---|
Bends | +/- 1 degree | +/- 1 degree |
Offsets | +/- 0.304mm | +/- 0.012 in. |
Hole Diameters | +/- 0.127mm | +/- 0.005 in. |
Hardware to edge/hole | +/- 0.254mm | +/- 0.010 in. |
Edge to edge/hole; hole to hole | +/- 0.127mm | +/- 0.005 in. |
Hardware to hardware | +/- 0.381mm | +/- 0.015 in. |
Bend to edge | +/- 0.254mm | +/- 0.010 in. |
Bend to hole/hardware/bend | +/- 0.381mm | +/- 0.015 in. |
Multiple Surface
Metric | US | |
---|---|---|
Features separated by two or more bends | +/- 0.762mm | +/- 0.030 in. |
Sheet Metal Material Thickness Range
\Sheet metal parts are formed from a single sheet of metal, requiring a uniform wall thickness throughout. Thickness typically ranges from 0.024 inches (0.609 mm) to 0.250 inches (6.35 mm).
Steel | Stainless | Aluminum | Copper | Brass | ||||||
---|---|---|---|---|---|---|---|---|---|---|
CRS/HRPO* | Galvanneal | Galvanized | 304-2B | 304-#4 | 316-2B | 5052-H32 | 6061-T6 | C101 | C110 | C260 |
0.024 | 0.028 | 0.028 | 0.024 | 0.024 | 0.024 | 0.025 | 0.025 | 0.025 | 0.025 | |
0.030 | 0.034 | 0.034 | 0.029 | 0.029 | 0.029 | 0.032 | 0.032 | 0.032 | 0.032 | 0.032 |
0.036 | 0.040 | 0.040 | 0.036 | 0.036 | 0.036 | |||||
0.042 | 0.040 | 0.040 | 0.040 | 0.040 | 0.040 | |||||
0.048 | 0.052 | 0.052 | 0.048 | 0.048 | 0.048 | 0.050 | 0.050 | 0.050 | 0.050 | 0.050 |
0.060 | 0.063 | 0.063 | 0.060 | 0.060 | 0.060 | 0.063 | 0.063 | 0.064 | 0.062 | 0.063 |
0.075 | 0.079 | 0.079 | 0.075 | 0.075 | 0.075 | 0.080 | 0.080 | 0.080 | 0.080 | 0.080 |
0.090 | 0.093 | 0.093 | 0.090 | 0.090 | 0.090 | 0.090 | 0.090 | 0.093 | 0.093 | 0.093 |
0.105 | 0.108 | 0.108 | 0.105 | 0.105 | 0.105 | 0.100 | 0.100 | |||
0.120 | 0.123 | 0.123 | 0.120 | 0.120 | 0.120 | 0.125 | 0.125 | 0.125 | 0.125 | 0.125 |
0.135* | 0.138 | 0.138 | 0.135 | 0.134 | 0.135 | |||||
0.164* | 0.165 | 0.160 | 0.160 | |||||||
0.179* | 0.187 | 0.190 | 0.190 | |||||||
0.239* | 0.250 | 0.250 | 0.250 |
*These thicknesses are available as Hot Rolled, Pickled & Oiled (HRPO) only
Surface Finishing
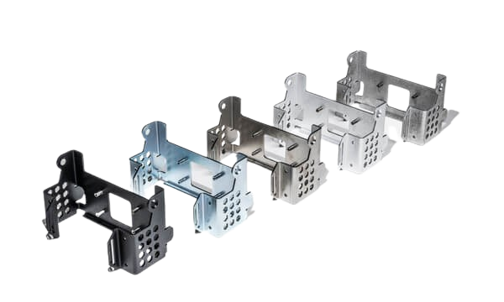
Design Guidelines for Bending
These guidelines are created to support product designers in understanding sheet metal fabrication and identifying the main factors that impact part quality and consistency. Applying this knowledge in your project can help control costs, meet visual expectations, and maintain high precision throughout production.
Brake Lines
Rake lines are a common result of the force applied to sheet metal during the bending process, typically performed using a press brake. These lines are inherent to the manufacturing process and will remain on the final product unless additional treatments are applied.
To remove these brake lines, a direct oscillating sanding device can be used. For parts requiring specific aesthetic qualities or a cosmetic finish, several secondary processing options are available:
-
Using a direct oscillating (DA) tool to blend the brake line into the surrounding material.
-
Placing a rubber slip sheet on top of the die tooling during the bending process.
-
Applying a finish, such as powder coating, after forming to cover the entire surface of the part.
Note: Plating thickness is often not sufficient to cover brake lines, so these lines may still be visible after plating.
If your part requires a uniform finish with no visible brake lines, please contact our applications engineers to explore the best method for your specific needs.
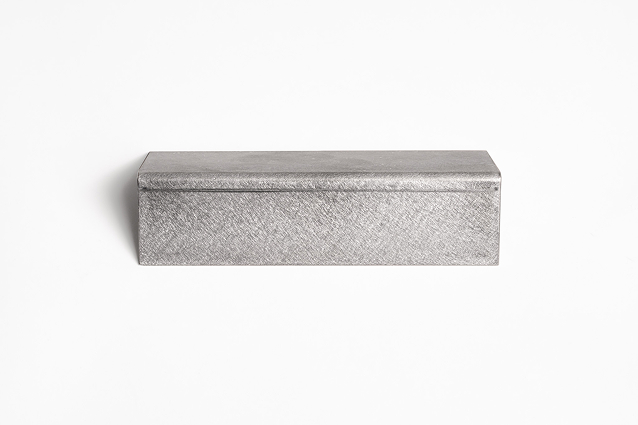
Bent sheet metal showing visible press brake lines.
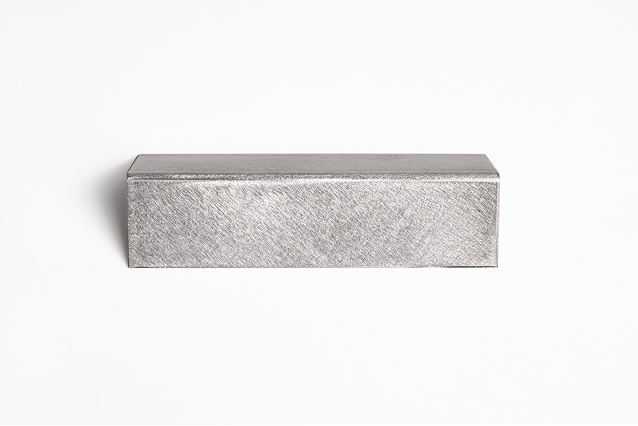
A direct oscillating sanding tool can effectively eliminate brake lines.
Feature Proximity
When sheet metal is bent in a press brake, the material naturally stretches along and near the bend line. Any feature located within a certain proximity of the bend—usually within 4 times the material thickness from the bend—is at risk of deformation.
However, there are several design strategies that can mitigate this issue and help you achieve your intended design outcome. Below are common design solutions for addressing this challenge:
Possible Solutions | Rationale for Using this Solution |
---|---|
Allow the feature to deform during bending | This solution is only recommended if deformation is acceptable, such as in cases where the feature’s criticality is low or during early prototyping. Future design changes can resolve the issue. Protolabs’ design for manufacturability analysis may suggest feature deformation as a viable option. Before manufacturing begins, our sheet metal fabrication experts will evaluate all potential deformation scenarios and contact you if any concerns arise. |
Change the bend radius | Opting for a smaller internal bend radius when features are close to, but not directly through, the bend can reduce material stretching at the bend. A smaller bend radius helps to minimize deformation risks. Consult our list of available internal bend radii to choose the best option for your design. |
Re-locate/move the at-risk feature | The most common solution when dimensional accuracy of the feature is essential. This approach involves repositioning the feature to a location where it is less prone to deformation. Our design for manufacturability toolkit provides a reference guide that outlines minimum feature distances based on material type and thickness, helping avoid distortion issues. |
In most cases, these design solutions do not significantly affect the cost or lead time of your project, but they can help avoid additional processing costs over the lifespan of your part.
Not sure which solution is best for your design? Our sheet metal experts are available to offer a free 30-minute design review, with same-day appointments available!
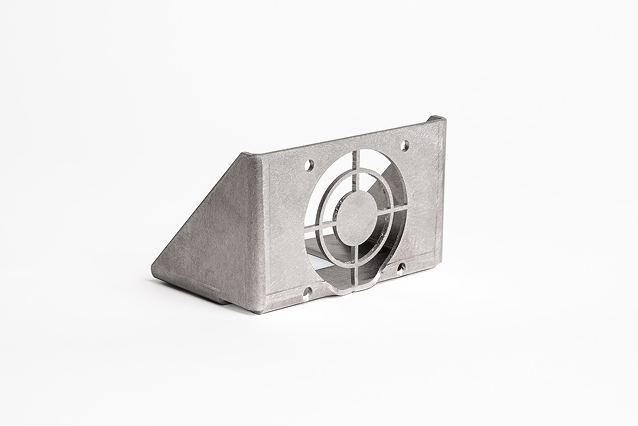
When features, such as the fan bracket and bottom holes, are too close to a bend, the sheet metal will experience deformation during the bending process.
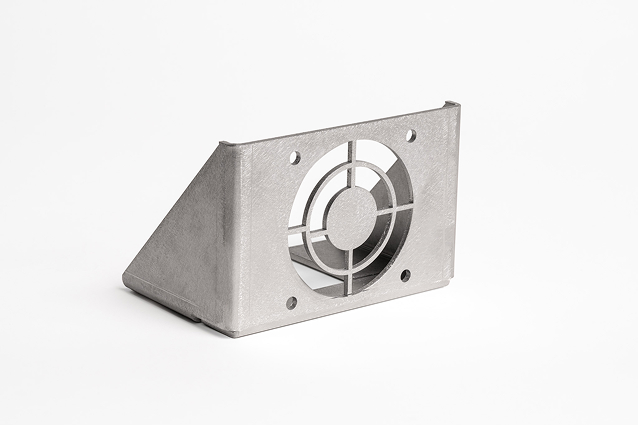
In this case, reducing the size of the internal bend radius effectively provided the extra material needed, preventing deformation of the fan cutout and holes.
Extending Features
One more option to prevent deformation along bends is to extend a feature through the bend.
In images A and B (below), the bracket has two L-shaped cutouts that terminate at the bend. The material experiences deformation where the top of each L intersects with the bend.
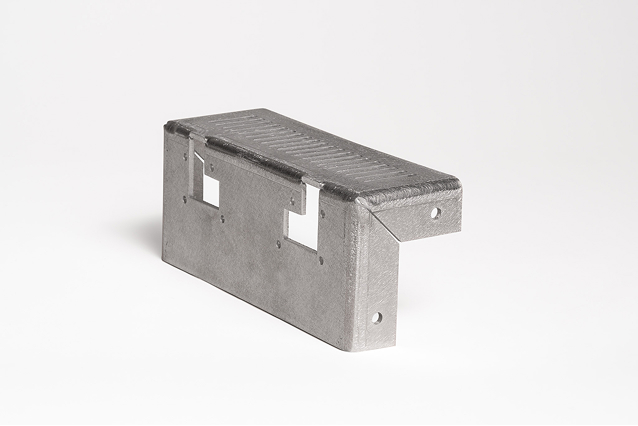
image A
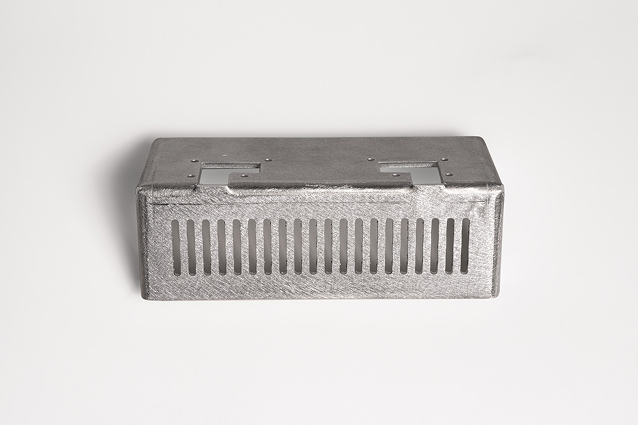
image B
By following the feature distance guidelines outlined in the “Placing Features Near Bends” section of our sheet metal DFM toolkit, deformation can be eliminated by extending the L-shape through the bend line. This method removes the material where the bend would typically occur, as demonstrated in images C and D below.
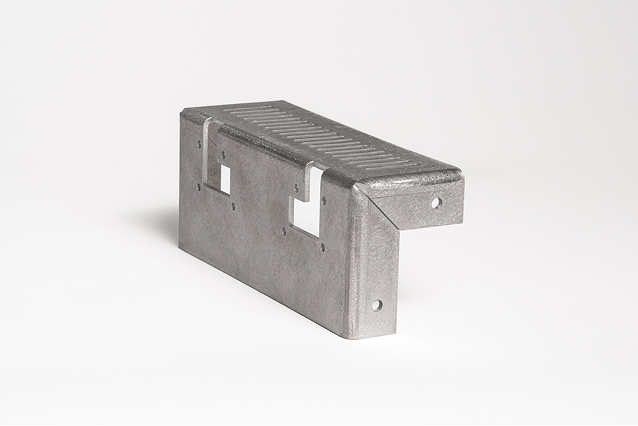
image C
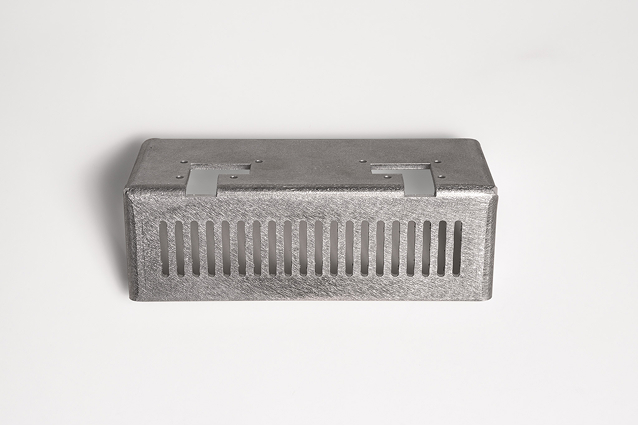
image D
Minimum Flange Lengths
To ensure an accurate bend in a press brake, the design must make three (3) points of contact with the machine. The image to the right highlights these crucial points of contact.
If only two points of contact are made, the final part may experience a deformed flange. For example, in the image below, the “H” bracket’s center portion has two flanges on the z-axis, which didn’t make three points of contact during the bending process. This resulted in inconsistent and deformed bends.
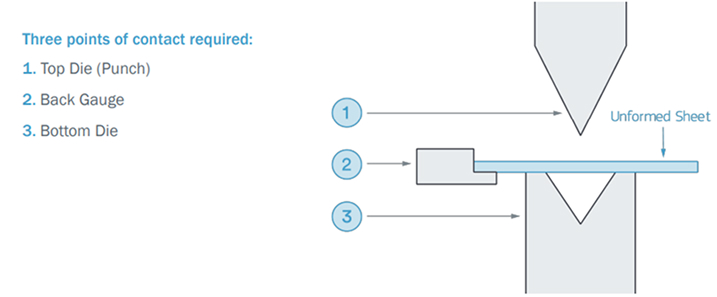
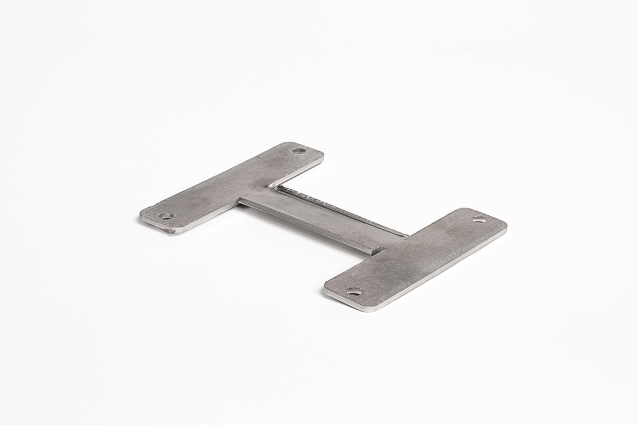
The center portion of this “H” bracket demonstrates quality issues due to insufficient material on the z-axis flanges, leading to bending inconsistencies.
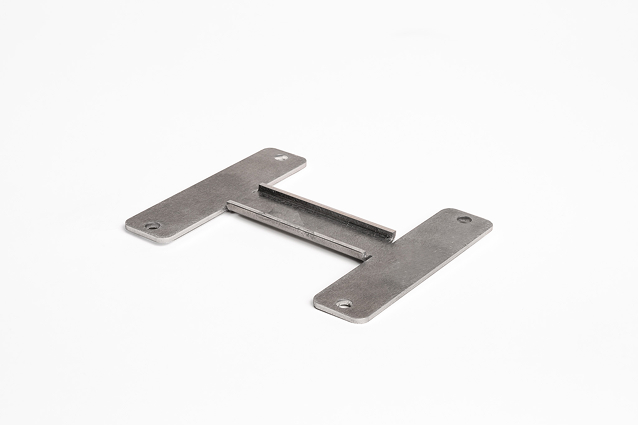
By adding material to a short flange, the workpiece can make three points of contact with the press brake, ensuring consistent and accurate bending.
Hardware Inserts
Including hardware inserts in your sheet metal design is an effective and affordable solution for joining components. However, when placing hardware holes and inserts near bend lines, it is crucial to account for potential deformation during the bending process.
If hardware holes are too close to a bend, the material will stretch, which may impact the integrity and functionality of the insert. The mounting bracket below demonstrates how the bending process can influence hardware positioning and installation.
To overcome this challenge, a recommended approach is to adjust the design by moving hardware holes further from the bend line or considering alternative insertion methods that ensure secure functionality even in challenging bending scenarios. This proactive design strategy can help avoid deformation and maintain the effectiveness of hardware inserts.
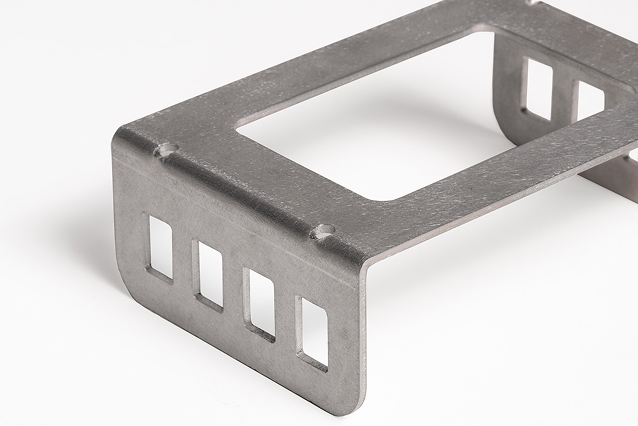
This mounting bracket has laser-cut holes along the bend line, causing the holes to become oblong (non-round) after forming.
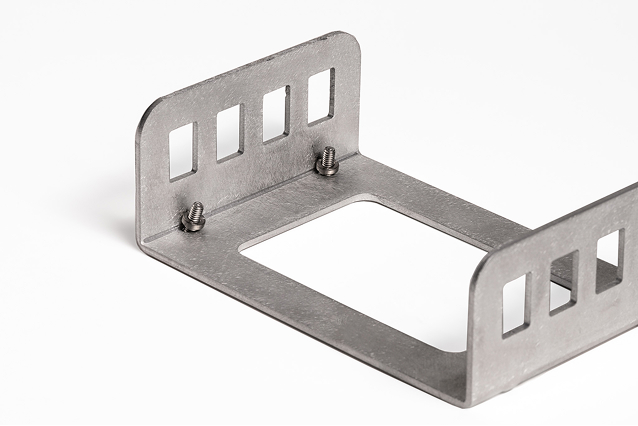
As a result, the hardware inserts won’t properly seat into the holes, preventing them from gripping the component material and impacting the functionality of the insert and the overall design.
The term “relief” is commonly used in sheet metal manufacturing, especially in the context of “bend relief,” but it can also apply to other design situations. Relieving sheet metal involves strategically placing cut-outs to enable material stretching during bending, preventing distortion of critical features.
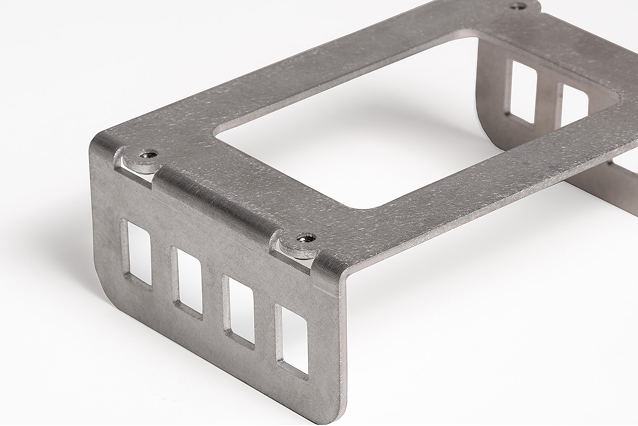
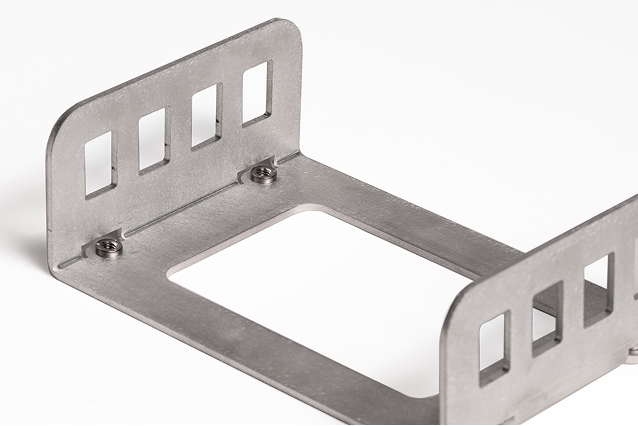
For hardware holes near bends, adding cutouts around the hole allows for material relief during bending, preventing distortion. This approach helps maintain the design intent while keeping costs low.
Considering material relief during the design phase offers several benefits, such as:
KingStar Mold’s Capabilities
Bend Radius
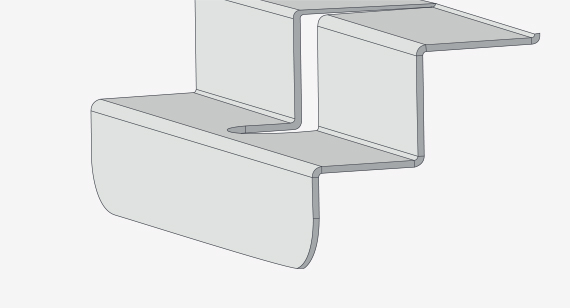
We maintain a tolerance of +/- 1 degree on all bend angles and offer a variety of common bend radii. Our standard options include 0.030 inches (0.762 mm), 0.060 inches (1.524 mm), 0.090 inches (2.286 mm), and 0.120 inches (3.048 mm), with tooling available on a 3-day lead time. For optimal results, it is advised to use consistent radii across all bends. Additionally, the minimum flange length for sheet metal parts must be at least four times the material thickness.
Hems
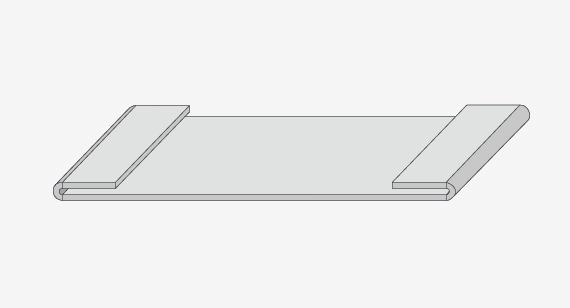
We produce both open and closed hems, with tolerances influenced by the hem’s radius, material thickness, and nearby features. For best results, we recommend a minimum inside diameter equal to the material thickness and a hem return length of six times the material thickness.
Offsets
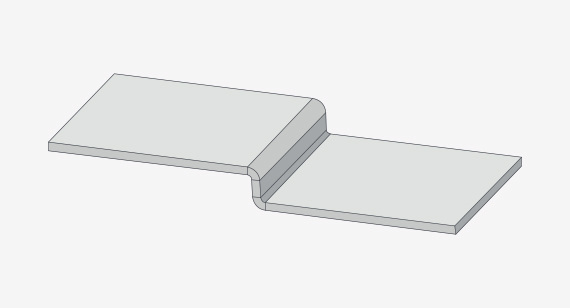
Holes and Slots
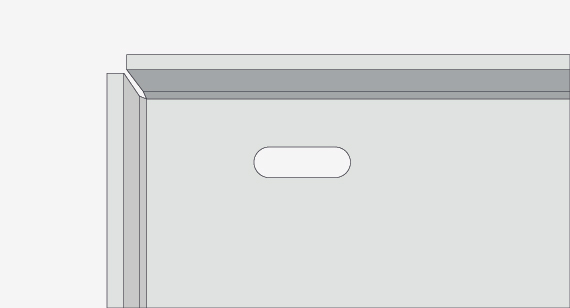
Notches and Tabs
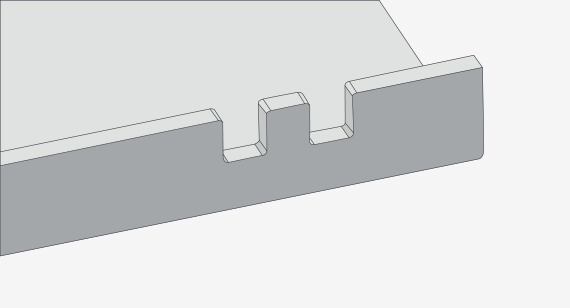
Notches should be at least the material thickness or 0.04 inches (1.016 mm), whichever is greater, and their length must not exceed five times their width. Tabs must measure at least twice the material thickness or 0.126 inches (3.200 mm), whichever is greater, with a maximum length of five times their width.
Countersinks
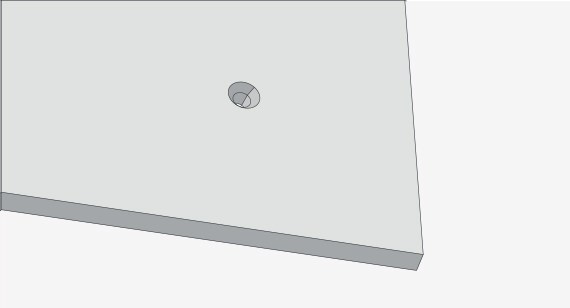
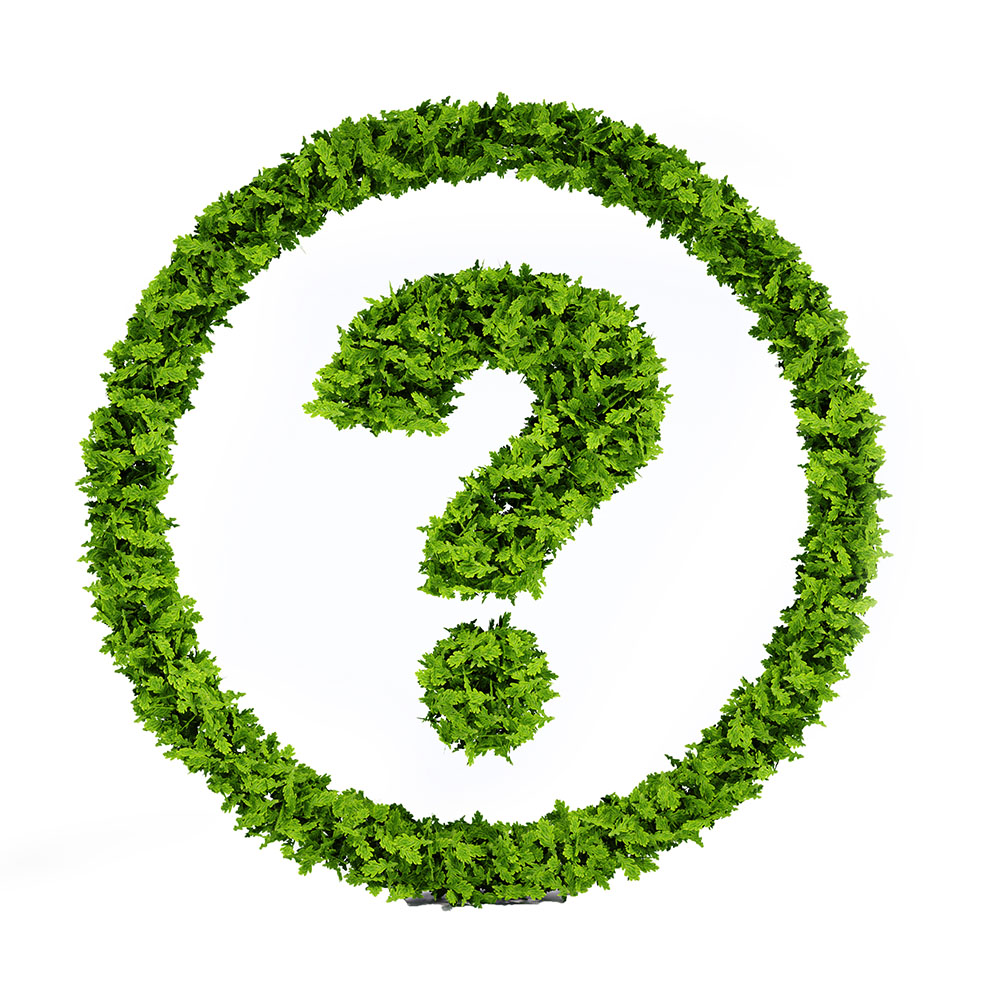