Fused Deposition Modeling (abbreviated as FDM) is one of the most widely used 3D printing technologies. It uses a heated nozzle to extrude thermoplastic filaments, depositing layers of material to build the entire object. Due to its simple operation, low cost, and strong adaptability, this method is highly popular among many enthusiasts, educators, and enterprises. FDM 3D printers are not only suitable for prototype production but also for practical applications, as they support various thermoplastic materials such as PLA, ABS, PETG, etc. Despite the development of other 3D printing technologies over the past decades, FDM still holds a considerable market share.
- 1. Brief Introduction of FDM History
- 2. How FDM Works
- 3. Inside FDM Printers: Key Hardware Setups
- 4. Performance Characteristics of Fused Deposition Modeling
- 5. Inherent Technical Constraints
- 6. Common Material Options for FDM Processes
- 7. FDM 3D Printer Options Comparison
- 8. Applications of FDM 3D Printing
- 9. FDM vs SLA vs SLS: A Quick Comparison
1. Brief Introduction of FDM History
Fused Deposition Modeling was invented in 1989 by Scott Crump as a faster, and more cost-effective way to create prototypes by extruding molten plastic layer by layer from digital designs. Later Crump and his wife founded a company – Stratasys, to commercialize the technology by releasing the first FDM machine in 1992. It’s initially used in industries like aerospace and automotive, then gradually expanded to other industries. Comparing to other traditional methods, FDM enabled rapid prototyping with less time and expense.
By the mid-2000s, FDM expanded into the consumer market through open-source projects like RepRap (an initiative aimed at creating a system where all components of a printer can be printed by a printer with same components to achieve “self-replication”) and affordable desktop printers from companies such as MakerBot. This shift made 3D printing accessible to hobbyists, educators, and small businesses, helping democratize manufacturing.
But that’s enough for history, let’s now turn to how FDM technology actually works.
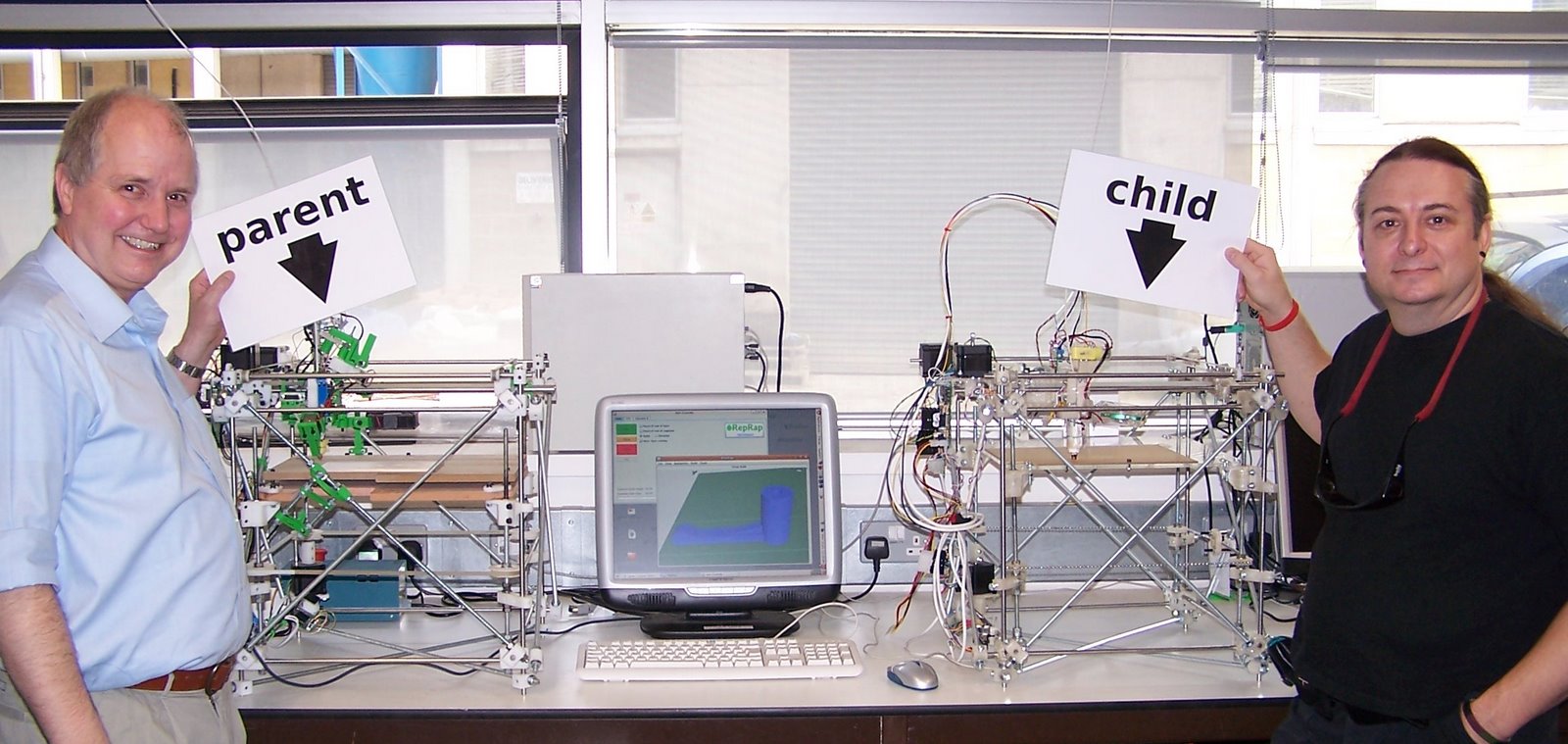
First Replication of Reprap Project from Wikipedia
2. How FDM Works
It is a 3D printing process based on material extrusion, where a heated nozzle deposits melted material onto a build surface layer by layer, fusing it together.
2.1 Material Extrusion and Layer-by-Layer Deposition
FDM works by feeding a thermoplastic filament through a heated nozzle that melts and deposits the material onto a build platform.
- Material Flow: As melted filament exits the nozzle, it rapidly cools and bonds with the previous layer, forming the object layer by layer.
- Layer Precision: This approach enables precise fabrication of complex shapes with minimal material waste.
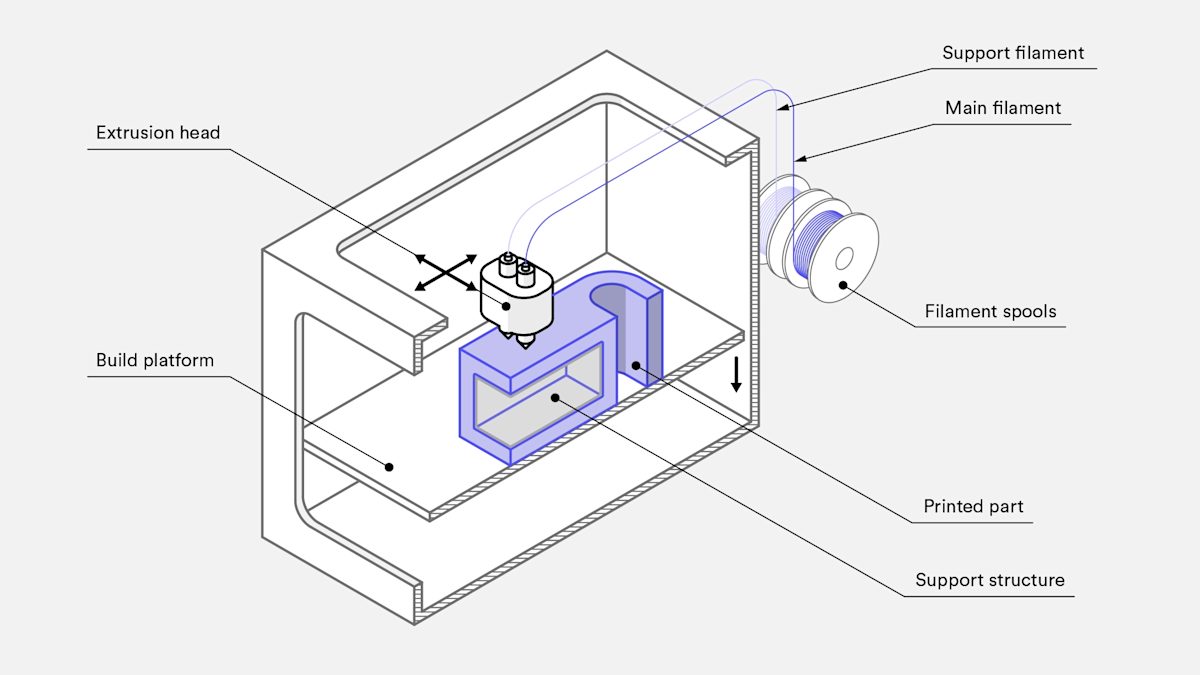
Illustration of FDM Printer Structure
2.2 Extrusion and Deposition System
The FDM system comprises two main components: the cold end and the hot end. These parts work together to feed, melt, and extrude the material in a precise manner to build the object layer by layer.
Cold End (Where Material is Fed)
The cold end is responsible for moving the filament from its spool to the hot end, where it will be melted and extruded. The cold end uses a motor-driven mechanism (often referred to as an “extruder”) to push the filament through a series of rollers or gears to ensure consistent feeding.
- Motorized Feed Mechanism: The cold end typically uses a stepper motor to control the speed at which the filament is pulled into the hot end. It ensures a steady, controlled flow of material, which is crucial for maintaining print quality.
• Filament Type: The cold end is able to adapt to different filament diameters (usually 1.75 millimeters or 2.85 millimeters) according to the printer model, providing users with the flexibility to choose from various filament types.
The precise movement and controlled delivery of the filaments are crucial for the success of the FDM printing process, as they ensure that the materials are delivered at the right time and in the right amount.
Hot End (Where Material Melts and Being Extruded)
The hot end is the key component of the entire device. This is where the filament being heated and extruded from the nozzle. It consists of a heating block, a thermistor (or a “heater”), and a nozzle, enabling the precise deposition of the melted material.
- Heating element: The heater inside the heating end melts the filament, and then the filament is pushed into the nozzle. The temperature is carefully controlled to ensure that the filament reaches the optimal viscosity, thus enabling smooth extrusion.
- Nozzle size: The diameter of the nozzle is usually between 0.2 millimeters and 1 millimeter. A small-diameter nozzle is used to ensure accuracy, while a large-diameter nozzle is used to increase the printing speed.
The hot end’s precision in melting and extruding the filament plays a major role in the quality of the printed object. Consistent temperature and material flow are crucial for successful prints.
2.3 Printhead Motion and the Movement Systems
The FDM printer employs various motion systems to control the movement of the nozzle and the deposition of materials according to different requirements. The choice of the motion system will affect many factors, such as the speed, precision, complexity, etc. of the printer.
Cartesian
The Cartesian system is the most common and straightforward approach used in FDM printing. It moves the printhead along the X, Y, and Z axes in a rectangular grid.
- Simple and Reliable: The Cartesian system is reliable and easy to control, making it popular for both entry-level and advanced 3D printers.
- Also, this setup allows for precise movements, ensuring accuracy in each layer of deposition.
Thus is a most used system for most desktop 3D printers (due to its simplicity and effectiveness).
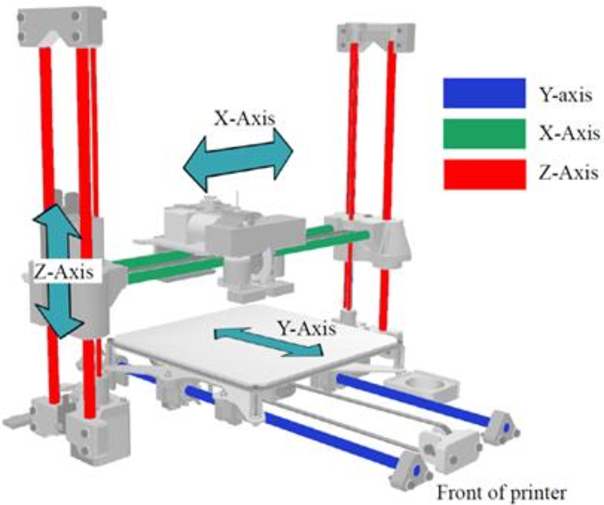
Cartesian Printer Illustration
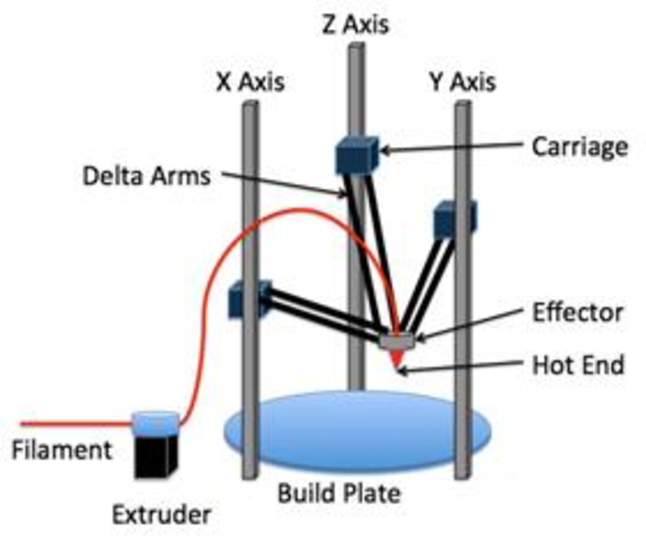
Delta Printer Illustration
Delta Systems: Speed and Agility
Picture three arms reaching down from vertical columns, moving the printhead in swift, triangular arcs. This is the Delta system, a favorite in printers built for speed and simpler geometries. What makes it stand out?
In terms of printing speed, the printer loaded with Delta system outperforms the traditional Cartesian-style printers. Its design directly converts the motor’s movement into the rapid movement of the print head, making it highly suitable for quickly creating prototypes.
Moreover, the unique geometric shape also brings practical benefits, providing extremely high precision – especially suitable for smaller and more complex models.
SCARA Arms: Precision for Specialized Tasks
While less prominent in consumer-facing 3D printing, SCARA (Selective Compliance Assembly Robot Arm) systems provide industrial-grade precision essential for specialized automation tasks.
- Articulated Motion Performance: SCARA arms excel in tasks requiring articulated kinematics, like manipulating at irregular orientations or placing components in assemblies with high precision. Rapid, highly repeatable motion is essential for these operations and is made possible by their architecture.
- Fundamental Mechanical Simplicity: SCARA configurations usually have a simpler mechanical design than other multi-axis motion systems. For applications that are well suited to its unique 4-axis motion profile, this transitions to a potentially lower total cost of ownership and less complicated integration, which are more friendly to users.
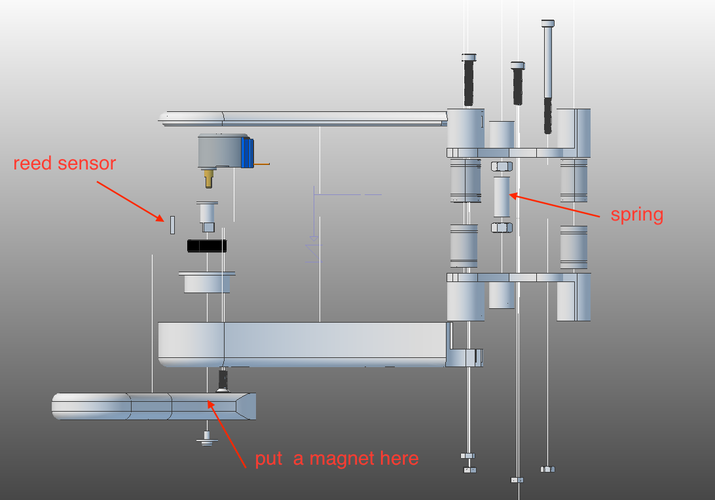
SCARA Printer Illustration
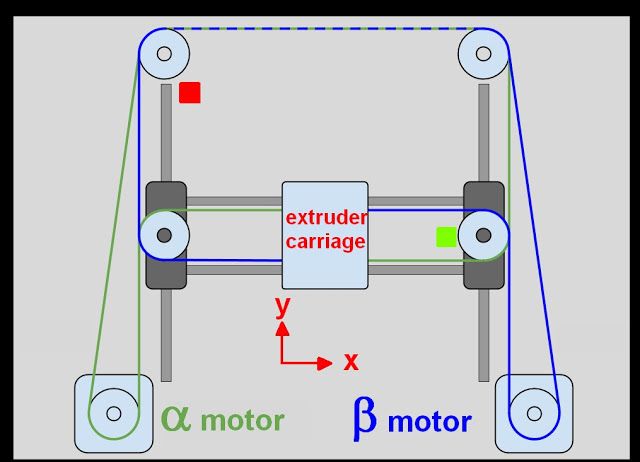
CoreXY Printer Illustration
CoreXY: Balancing Speed and Detail
CoreXY’s movement is unique. The axes of X and Y are controlled by two motors that cooperate via a clever belt-and-pulley configuration. This synergy improves its performance.
It is well known for moving quickly across the print bed. When compared to many other designs, this drastically reduces the overall print times. However, speed does not necessarily come at the expense of details. For professional and industrial printers where both speed and precision are crucial, CoreXY is a powerful choice as it maintains excellent accuracy even when moving rapidly. It is the preferred system in demanding environments.
3. Inside FDM Printers: Key Hardware Setups
The magic of FDM printing hinges on its hardware. How components like the filament pusher (extruder), the melting chamber (hot end), and the movement system work together defines a printer’s capabilities – its speed, precision, and what materials it can handle. Let’s break down the critical hardware configurations.
3.1 Direct Drive vs. Bowden: Pushing Filament
Getting filament smoothly to the melt zone is crucial. Two main approaches dominate:
Direct Drive: Control Up Close
- How it Works: The motor pushing the filament sits right on the printhead. Filament goes straight into the hot end, no detours.
- The Upside: This direct path gives superb control, especially with soft, flexible filaments like TPU. Less chance of the filament kinking or slipping means more reliable printing with tricky materials.
- The Trade-off: Putting that motor on the moving head adds weight. This can slightly limit top speeds and make super-precise, high-frequency movements a bit harder to achieve.
Bowden Systems: Keeping it Light
- How it Works: The extruder motor stays fixed on the printer’s frame. A long tube (the Bowden tube) guides the filament down to the distant hot end on the moving head.
- The Upside: With less mass to shuffle around, the printhead moves faster and with potentially greater precision, a real advantage for large prints.
- The Trade-off: That long tube is the weak spot for flexible filaments. They can bend, buckle, or compress inside the tube before reaching the hot end, leading to printing issues.
3.2 Hot End Choices: Melting it Right
The hot end is where the plastic turns liquid. The materials used inside it define what you can print:
All-Metal Hot Ends: High-Temperature Powerhouses
- Built Tough: Every part touching the hot filament is metal – no plastic liners inside the critical heat zone.
- Why Choose One: They handle serious heat (easily 450°C+) needed for advanced materials like Nylon, ABS, or Polycarbonate. They’re also generally more durable over the long haul.
- Considerations: Running hotter demands better cooling to prevent jams (“heat creep”). They can be slightly more finicky to tune initially and might wear faster with abrasive filaments.
PTFE-Lined Hot Ends: Smooth & Simple for Standard Plastics
- The Design: A slick PTFE (Teflon®) tube lines the path inside the upper part of the hot end (“heat break”), guiding filament smoothly right down to where it melts.
- Why Choose One: They’re incredibly reliable and easy to use with common filaments like PLA and PETG, offering smooth feeding and fewer clogs out-of-the-box. Setup and maintenance are usually simpler.
- The Limits: That PTFE liner starts breaking down around 250° This rules out high-temp materials and means abrasive filaments (like glow-in-the-dark or carbon fiber filled) will chew up the liner much faster. It’s a consumable part.
Choosing the right setup boils down to your needs: What materials do you need to print? How critical is raw speed versus handling flexibility? Understanding these hardware choices is key to picking or tuning your FDM machine.
3.3 Multi-Extrusion Setups for Multi-Material Printing
Want to print objects combining rigid frames with rubber-like grips? Or create full-color prototypes straight off the build plate? That’s where multi-extrusion systems shine. These setups equip printers with multiple extruders and hot ends, letting you work with different filaments in a single print job – whether for functional composites or vibrant visual effects.
Dual Extrusion: Dual extrusion setups typically use two extruders and hot ends, allowing for two different materials to be printed in one object. For example, one material might be used for the main structure, while the second material could be used for support structures that are easier to remove.
And then there’s Multi Extrusion:Stepping up to three or more extruders unlocks even wilder possibilities, but demands serious calibration:
- Complex Material Cocktails: Blend plastics with contrasting properties in one object – like combining:
- High-strength components
- Flexible hinges
- Conductive traces
- Water-soluble internal supports
- True Color Printing: Achieve photorealistic models by laying down 4+ colored filaments simultaneously– no post-processing painting required
The Trade-offs (Because Nothing’s Perfect)
More extruders mean more complexity:
- Weight & Speed: Additional hot ends slow down printhead movement
- Oozing Challenges: Idle nozzles can drip and contaminate prints
- Precision Demands: Nozzles must be perfectly leveled to avoid collisions
- Material Juggling: Some filaments (like PETG and TPU) shouldn’t share the same hot end temperatures
Still, when you need that perfect blend of artistic flair and engineering functionality, multi-extrusion is the best choice of all. Just expect to spend extra time tuning retraction settings and nozzle alignments!
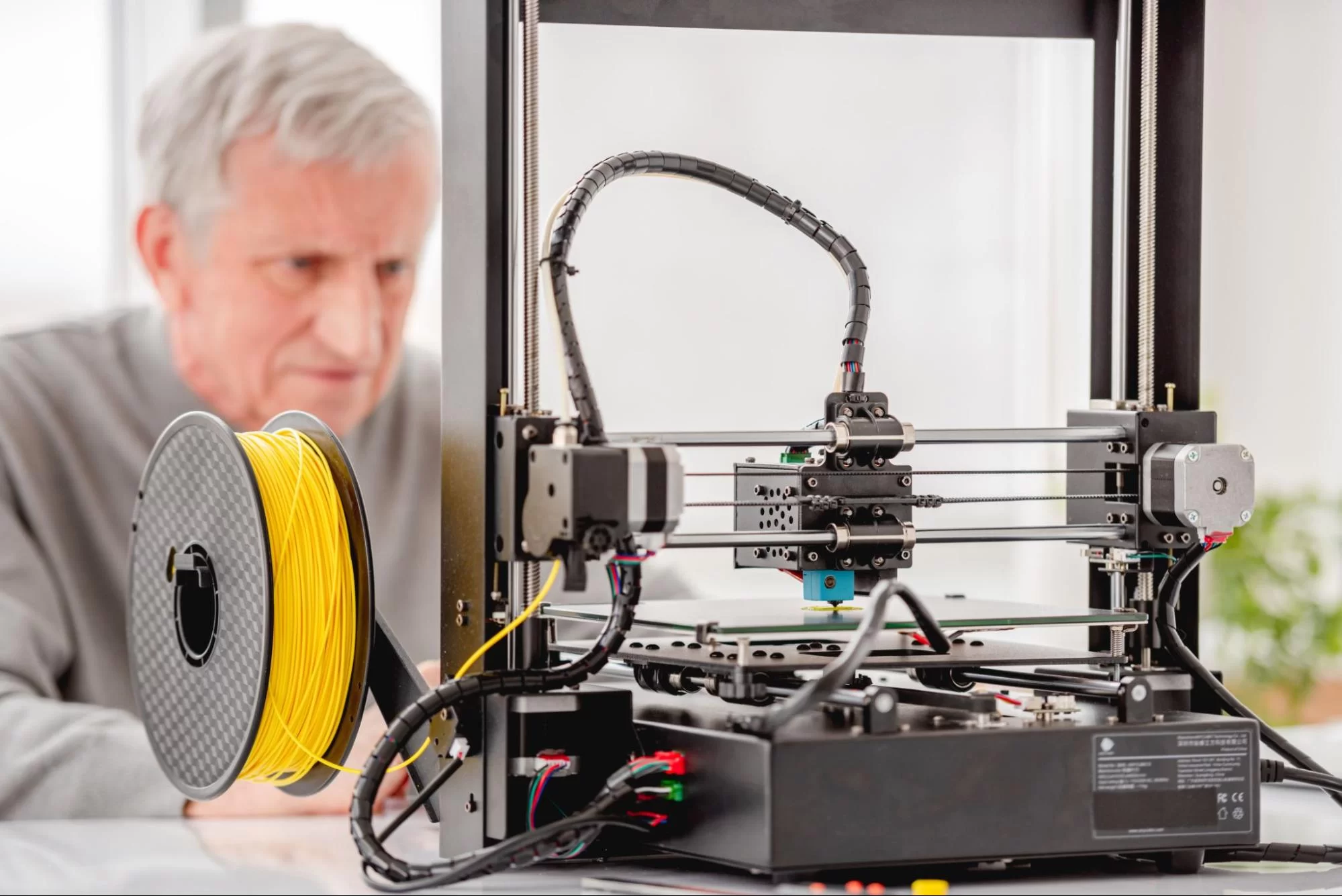
4. Performance Characteristics of Fused Deposition Modeling
FDM technology delivers distinct operational advantages that explain its dominance across prototyping, educational, and low-volume manufacturing contexts. These attributes merit consideration when selecting additive manufacturing processes.
4.1 Economic and Scalability Factors
The technology demonstrates compelling cost efficiency relative to photopolymer or powder-bed systems. Consider that thermoplastic filament commodities remain substantially more economical than specialized resins or metal powders. Hardware acquisition costs have undergone progressive deflation – quality systems now accessible at under $500 USD. Crucially, the architecture scales without fundamental reconfiguration: identical equipment produces singular prototypes or batch quantities exceeding 1,000 units. Educational institutions particularly benefit from this financial accessibility.
4.2 Material Adaptation Capabilities
Material versatility constitutes a principal advantage. The filament portfolio spans commodity plastics (PLA, ABS) to engineering-grade polymers (PC, nylon) and specialty composites (carbon-fiber reinforced, dissolvable). Selection criteria should address mechanical stress profiles, thermal thresholds, and chemical exposure – for your information, detailed material properties are in Section 6.
4.3 Operational Accessibility
Simplified workflows distinguish FDM from alternative processes. The extrusion mechanism requires neither controlled atmospheres nor specialized facilities. Most systems operate effectively within standard office environments. Software integration has matured considerably: contemporary slicers automate complex path planning while retaining granular parameter control when required.
4.4 Post-Processing Efficiency
Finished components typically require only support structure removal. Unlike resin-based outputs demanding chemical baths and UV curing, FDM parts achieve functional readiness immediately post-build. Minor finishing (sanding, acetone smoothing) remains optional rather than mandatory.
5. Inherent Technical Constraints
Certain limitations necessitate strategic process planning:
Maintenance
- Bed leveling must be checked regularly.
- Nozzles should be cleaned every 200–500 hours.
- Motion parts need lubrication to maintain print quality.
Interlayer Adhesion
- Vertical strength is weaker due to layer-by-layer bonding.
- Controlled temperature and slower speeds improve bonding.
Resolution Limits
- Print resolution is tied to nozzle size (≥2mm).
- Lower layer heights below 0.05mm often yield diminishing returns.
- Layering causes visible “stair-step”
Filament Quality
- Diameter variation > ±03mm affects extrusion.
- Moisture-sensitive filaments like nylon must be dried.
- Abrasive filaments wear nozzles faster.
6. Common Material Options for FDM Processes
As one of the most widely used 3D printing method, its wide material options support diverse applications:
Standard Polymers: PLA (easy to print), ABS (impact resistant), PETG (chemically durable)
Engineered Composites: Carbon/glass-filled for stiffness, TPU for flexibility, conductive blends for electronics
High-Performance Thermoplastics: PC (110°C+), PEEK (250°C+, aerospace and medical use)
Sustainable Materials: Recycled PET, biodegradable PLA blends
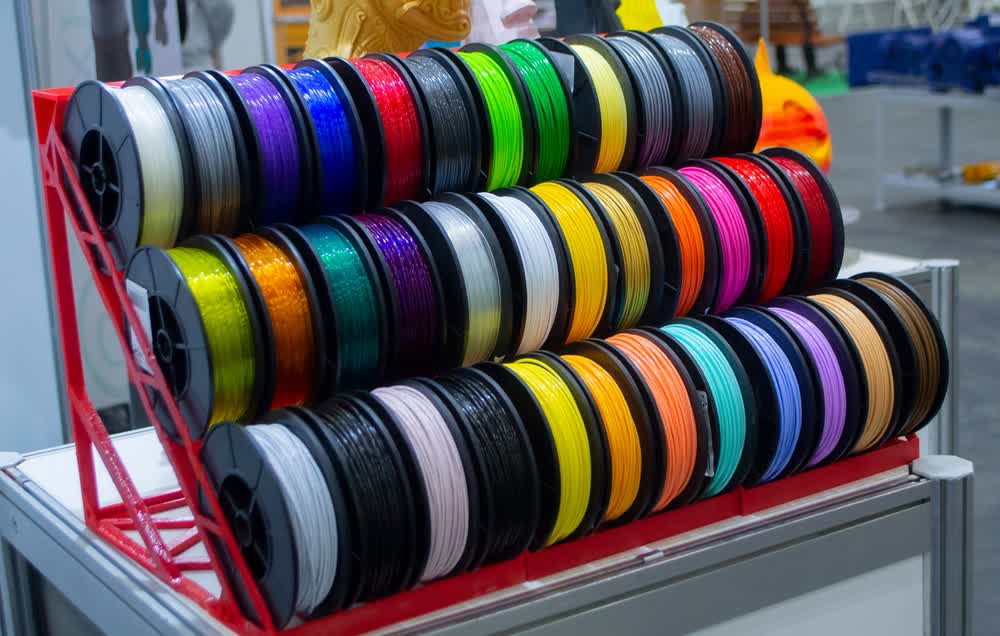
7. FDM 3D Printer Options Comparison
Category | Budget FDM Printers | Mid-Range FDM Printers | High-End FDM Printers |
Price Range | Below $500 | $500 – $2,000 | Above $2,000 |
Target Audience | Hobbyists, educational use, beginners | Advanced hobbyists, small businesses | Professionals, industrial use, businesses requiring precision |
Print Quality | Good for basic models, lower precision | Higher precision, good for detailed prints | Excellent print quality, highly detailed, consistent results |
Material Compatibility | PLA, PETG, some ABS | PLA, ABS, PETG, TPU, flexible filaments, carbon fiber-infused | PLA, ABS, PETG, Nylon, TPU, PVA, carbon fiber-infused, and more |
Build Size | Small (typically <200mm x 200mm x 200mm) | Medium (around 250mm x 250mm x 250mm) | Large (up to 400mm x 400mm x 400mm and beyond) |
Features | Manual bed leveling, single extruder | Auto bed leveling, touchscreen, dual extrusion options | Auto bed leveling, dual extrusion, high precision, touchscreen |
Speed and Reliability | Moderate speed, reliability varies | Faster and more reliable than budget options | High speed, highly reliable for long print runs |
Maintenance | Requires frequent calibration and maintenance | Moderate maintenance needs, easier calibration | Low maintenance, designed for high-volume use |
Examples | Creality Ender 3, Anycubic i3, Artillery Sidewinder X1 | Prusa i3 MK3S, Anycubic Mega S, Ender 5 Plus | Ultimaker S5, Raise3D Pro2, MakerBot METHOD X |
8. Applications of FDM 3D Printing
FDM 3D printing is widely adopted across industries, for example
- Prototyping & Product Development: Engineers use FDM to rapidly iterate designs, reducing development time and cost
- Functional Parts and Tools: Custom brackets, jigs, and enclosures are frequently printed for end-use or production support
- Hobbyist or Educational Projects: DIY makers, students, and educators rely on FDM for custom gadgets, models, and hands-on learning
- Automotive & Engineering: Used for mockups, test fixtures, and even semi-structural parts in custom builds
- Consumer Products: Small businesses print cases, accessories, and bespoke goods directly for sale or use
9. FDM vs SLA vs SLS: A Quick Comparison
Feature | FDM 3D Printing | SLA 3D Printing | SLS 3D Printing |
Material | Thermoplastics | Resin | Nylon powder |
Surface Finish | Moderate | Very Smooth | Moderate |
Strength | Good in-plane | Brittle | Strong overall |
Detail Level | Moderate | High | High |
Cost | Low | Medium | High |
Best Use | Prototypes, functional parts | Miniatures, models | Industrial parts |
Fused Deposition Modeling (FDM) continues to be a cornerstone of modern 3D printing, offering an ideal balance of affordability, material flexibility, and ease of use. From early-stage prototypes to end-use parts, FDM empowers users across industries to innovate faster and more efficiently. Its accessibility has opened the door for everyone—from hobbyists to engineers—to bring ideas to life with minimal barriers.
At KingStar Mold, we offer professional FDM as well as other 3D printing services tailored to your specific needs. Whether you’re developing a concept model or producing functional parts, our advanced equipment and engineering expertise ensure high-quality, cost-effective results. Let us help you turn your designs into reality with reliable FDM solutions backed by manufacturing excellence.